Standards have great impact on any industry since quality of standards determines quality and stability of products and ultimately customer confidence in these products. As such, it is fair to state: “no standard – no business”. IEC TC 36, the Standards Committee responsible for insulators, is tasked with developing standards that satisfy market needs. This is done using a Strategic Business Plan that is reviewed and maintained periodically to assure ‘continuous improvement’ in the face of new technologies and as well as changes in environmental, safety and other requirements.
The insulator industry is by nature highly conservative. In the electronics sector, product life cycles are often measured in months; in the insulator industry, by contrast, these are measured in decades. Still, this industry must also allow room for entry of innovative designs and concepts. This is where standards play a fundamental and decisive role. Jens M. Seifert of Germany, Chairman of IEC Technical Committee (TC) 36 ‘High-Voltage Insulators’, reviews key changes and developments in insulator standards over the past 25 years. He also presents some of the important activities now taking place within this Committee.
Scope & Structure
Scope of TC 36: Insulators
Standardization of insulators for high voltage systems and equipment including bushings, insulators for overhead lines, substations and their couplings.
Scope of SC 36A: Insulated Bushings
Standardization of insulated bushings and of couplings of these insulators.
TC 36 was established in June 1949 to deal with high voltage testing, wall bushings and insulators. In 1955, high voltage testing was assigned to the newly created TC 42 and the scope of TC 36 became preparing IEC recommendations on bushing insulators, line insulators and related equipment. In 1966, TC 36 was re-organized and three Sub-Committees were established: Sub-Committee A – Insulated bushings; Sub-Committee B – Insulators for overhead lines; and Sub-Committee C – Insulators for substations.
The scope of TC 36 was revised yet again in 1998 to become: standardization of insulators for high voltage systems and equipment including bushings, insulators for overhead lines and substations and their couplings. The field of activity of each Sub-Committee then applied to the specific types of insulators that fell within its scope. The technical activity of the parent Committee, by contrast, focused on preparation of international standards on topics common to insulators for different applications such as bushings, overhead lines and substations. This included generalized test methods (e.g. artificial pollution tests and power arc tests), guidelines for selection of insulators under polluted conditions, etc. In 2013, Sub-Committees B and C were consolidated once more into TC 36.
Standards for Composite Insulators
Twenty years ago, there were IEC, ANSI, VDE as well as numerous other national standards for insulators. At the time, standards for ‘classical’ insulators made of glass or porcelain (e.g. IEC 60383, 60233 and 60168) were already mature and widely accepted by manufacturers, customers and test institutes. In 1992, the first IEC standard for composite insulators was published (IEC 61109) and this was similar in many respects to ANSI C29.11, published three years earlier.
If one considers that the basic design principle for composite insulators was developed in 1965, the more than 25 years needed to issue the first applicable IEC standard seems unusually long. This is explained, however, by the fact that composite insulators were initially totally unknown. There was some experience with polymeric cast epoxy insulators but this was even more limited in the case of outdoor applications. Moreover, during the 1970s there were only a handful of composite insulator manufacturers and these suppliers dealt mainly with several ‘test customers’ – European and U.S. utilities that evaluated these innovative products in pilot installations from 110 kV to 765 kV. The potential advantages offered by his technology, such as light weight, high strength and resistance to dynamic impact from gun shot or during load transposition were all highly appreciated. In fact, these products were sometimes marketed as ‘miracle insulators’ and parallel trends favoring synthetic materials in many other industries only reinforced initial market interest.
Standards must be based on experience and best practice. Yet neither was available at the time composite insulators first began to be applied. Since early users naturally asked for specifications and guidance to support their application decisions, a growing body of technical knowledge was progressively developed, collected step-by-step and then eventually compiled into new standards. Some examples:
• The water diffusion, tracking & erosion and low voltage power arc resistance (material) tests were established in 1982 in German Standard, VDE 0441. In fact, this was the first standard for composite insulators. These tests were selected from other VDE standards applying mainly to cast epoxy materials. Acceptance levels were calibrated to an expected life of 15 years – the longest service experience at the time – in terms of minimum requirements. The basic philosophy was that if an insulator passed this test, a life expectancy of at least 15 years could be assumed for its material in an outdoor application. Later on, these same tests and acceptance levels were further refined and eventually incorporated into IEC 61109:1992.
• In regard to load-time curves, it was initially believed that composite insulators have high short-term strength but then lose strength over time if stressed at different mechanical load-time points, as shown in Fig. 1. This diagram was derived from a theoretical slope assumption that resulted in a 33% SML load capability at a 50-year load-time – then regarded as the maximum working load over a 50-year service life.
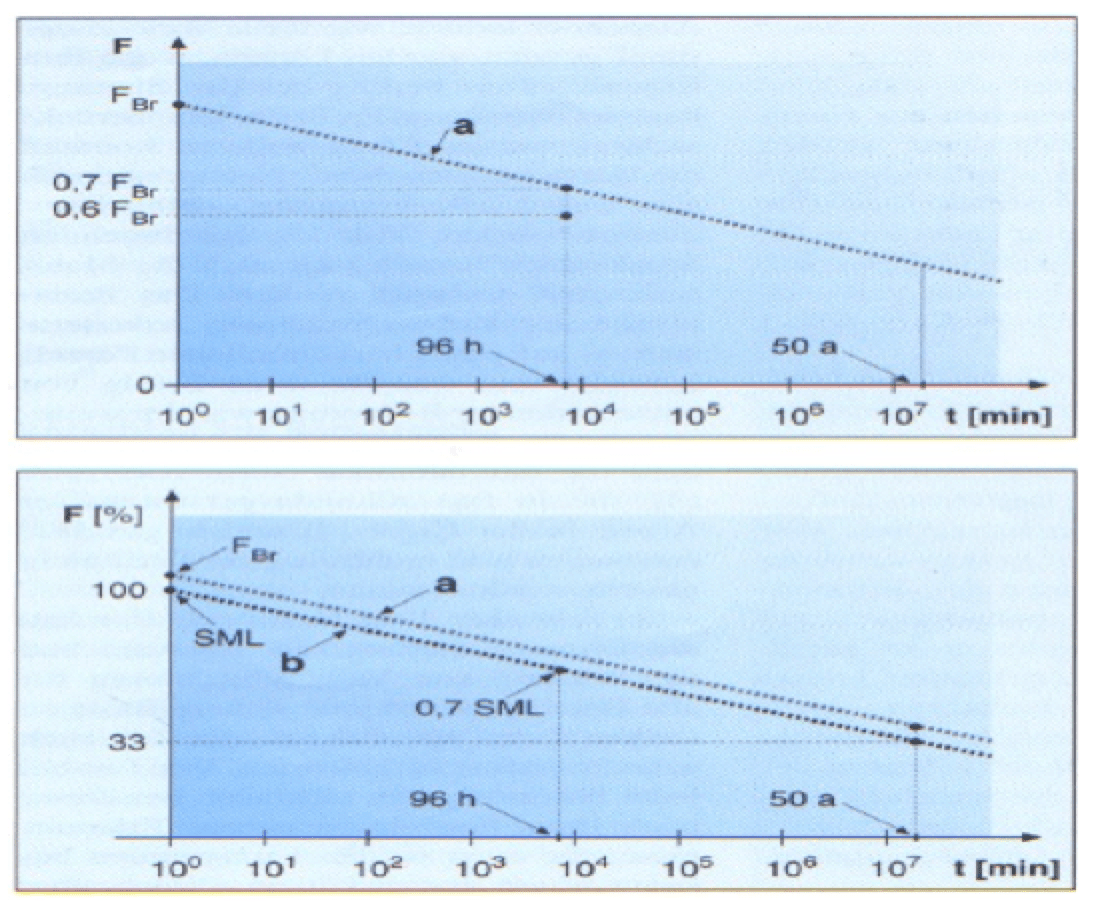
CLICK TO ENLARGE
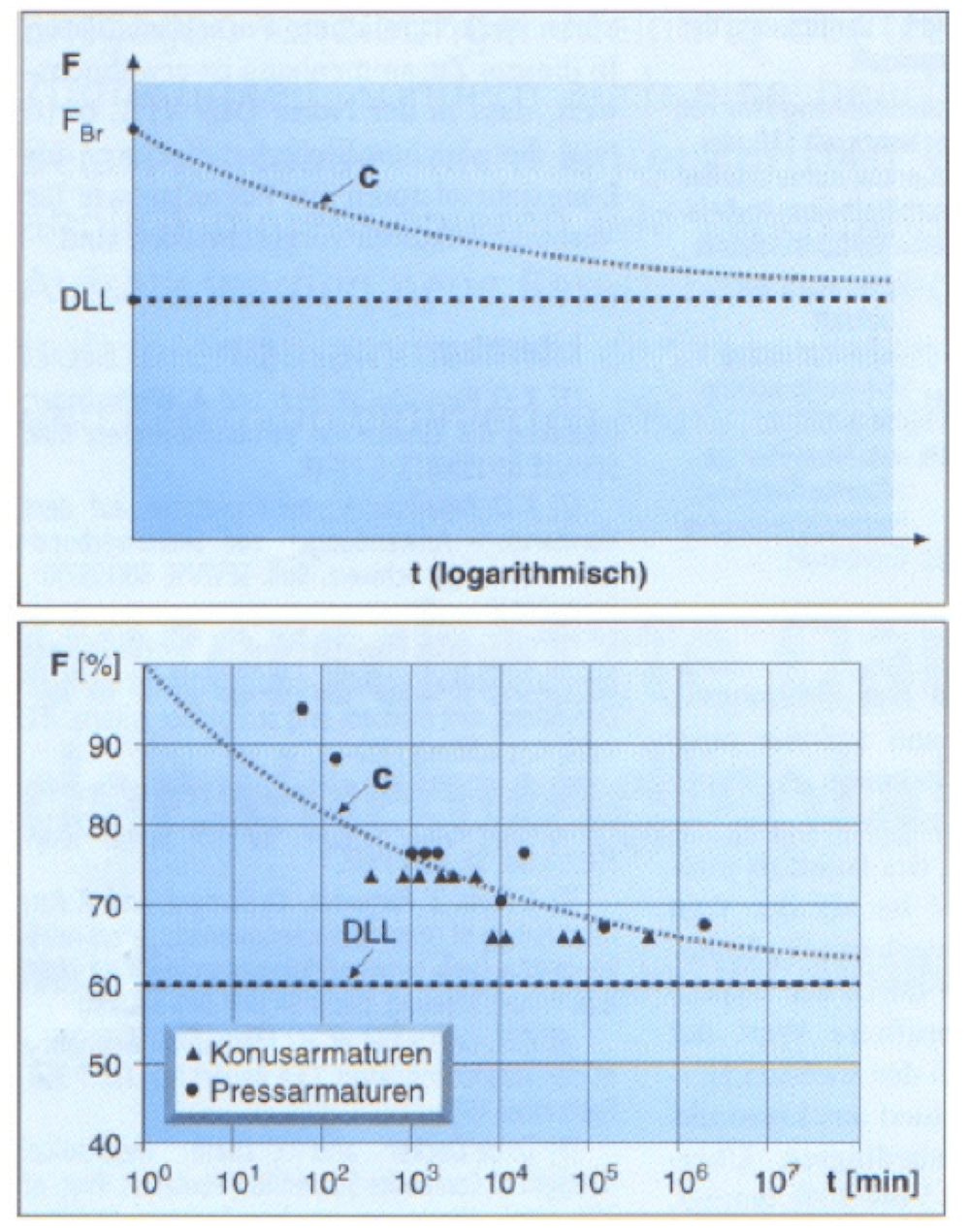
CLICK TO ENLARGE
Unfortunately, the charts in Fig. 1 created some misconceptions. For example, many users assumed from these curves that composite insulators lose strength over time, even though this is not true. With Edition 2 of IEC 61109:2008, user understanding improved due to introduction of the philosophy of ‘Damage Limit Level (DLL), as shown in Fig. 2. Tests and service experience confirmed that the mechanical lifetime of composite insulators seems infinite as long as permanent load remains below some critical DLL. In the case of most designs, this falls between 60 and 80% of the SML but depends greatly on how tight a margin was calculated during design. There is ongoing debate about the correct interpretation of load-time curves as well as the role of SML as the characteristic value for all relevant type and design tests in relation to ‘nominal load’.
This experience demonstrates that standards must be written in a comprehensive manner and may also need to offer additional practical guidance to users, such as in the form of application guides. Indeed, this past deficiency in regard to load co-ordination and performance of mechanical components triggered the recent decision to revise IEC 61109: Edition 3.
After 1992, there was much effort to develop new standards for all types of composite insulators and this resulted in IEC 61466:1998 for overhead line composite insulators, IEC 61462:1998 for composite hollow core insulators and IEC 61952:2002 for composite line post insulators. The work behind these standards was handled mainly by WG 10 of CIGRE SC 36B – a group of experts that included Clive Lumb, Ray Houlgate, Wallace Vosloo, Claude de Toureil, Alberto Pigini, K. Naito, Martin Kuhl, Richard Martin and Igor Gutman, among others.
The relatively intense standardization phase that took place starting in the late 1990s reflected industry need for standards that apply to any innovation that has entered the stage of market trial and growing acceptance (see Fig. 3). In fact, defining common terms, test methods and acceptance criteria for composite insulators is what allowed users to issue purchase specifications and requirements and therefore paved the way to broader application of this technology. This market acceptance phase was also characterized by a shift in industry scale – from mostly smaller pilot plants to large-scale factories that feature high volume production with systematic quality control processes at every step.
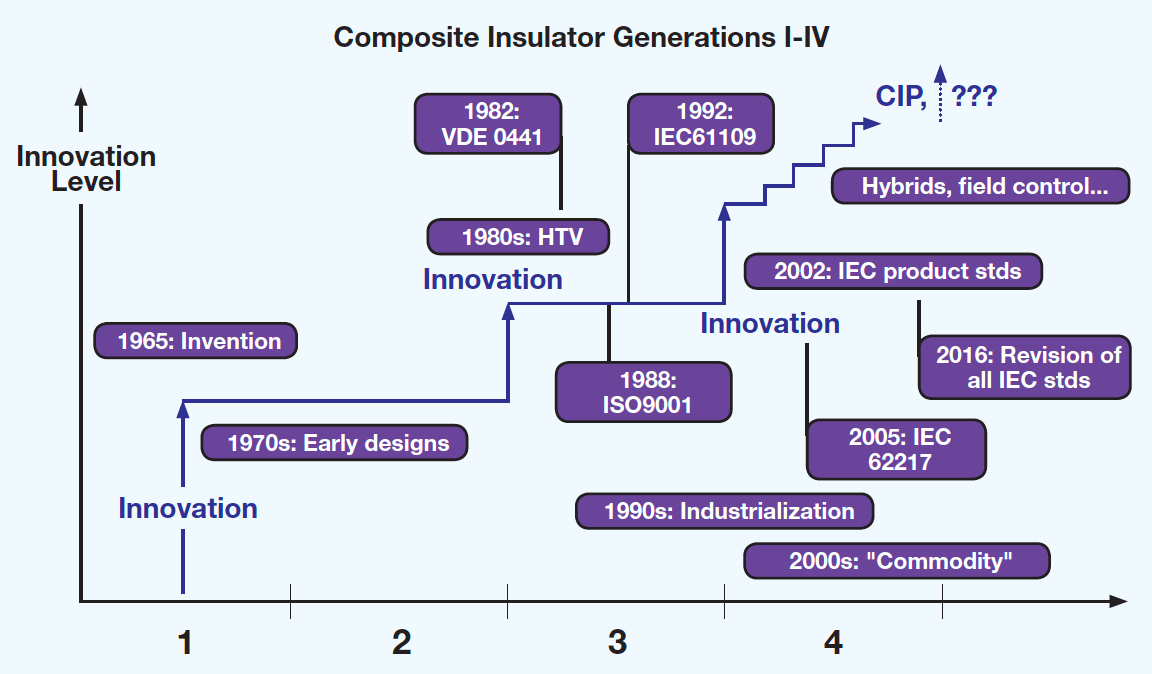
CLICK TO ENLARGE
In spite of the absence of product standards, user acceptance of composite insulators was comparatively high during their launch in the 1970s and 80s. But interest then declined due to concerns about possible premature ageing combined with reports of problems with some first and second-generation designs. Professor Hermann Kärner, Chairman of TC 36 from 1994 to 2000 consolidated these issues into a matrix that summarized how design and quality of materials each impact insulator performance. He then pushed for better standards for insulator designs as well as for more stringent material tests. In reaction, the TC 36 General Meeting in Stockholm in 2000 decided that a common clause standard for polymeric insulators must be developed to improve and simplify costly design testing, such as accelerated ageing tests, and rather to cover all design tests within a single IEC document (under common clauses). These design tests would then be valid for all different types of insulators (e.g. long rods, hollow cores, posts) that have a common basic design or technology. This task was later taken over by WG 12 (disbanded in 2016), which published IEC 62217 Ed 1.0 in 2005 and other standards such as IEC TR 62662, IEC TR 62730 and IEC TS 62896. With the next revision of IEC 62217:2012, ageing tests were discussed further and eventually separated from this document. Professor Josef Kindersberger, Chairman of TC 36 from 2004 to 2014, insisted on maintaining the IEC principle of “only one test for one property”. As a result, the wheel test and the 5000h test both had to be removed from IEC 62217 and instead were documented in IEC TR 62730. Both were considered as tracking and erosion design tests.
With revision of IEC 62217:Ed. 3.0 in 2016, the 5000h test came back as an ageing test with modified test conditions. This was due to market demand for a cyclic ageing test. At the same time, a framework was developed for the family of IEC 60815 standards for AC and DC covering classical as well as composite insulators. The entire project required 16 years before final publication of IEC 60815-4 and raised the question whether the TC 36 standardization process might be too slow, especially given the rapid re-emergence of HVDC technology over the past 2 decades.
During the 2000s, composite insulators came to be regarded more and more as a commodity and the number of manufacturers spiraled from only a few in the 1980s to well over a 100 today. Moreover, by 2006, IEC standards for composite insulators were already fully developed with available test and product standards that, while perhaps not perfect, are still sufficient and mature enough to serve market needs. It should also be noted that development of standards for classical ceramic insulators made of glass and porcelain basically ceased in 1993 with the latest revision of IEC 60383:1993. One could well ask whether or not there was need for improved standards to govern the quality of these products as well.
The task of TC 36 for the future is to improve standards in terms of a continuous improvement process (CIP) – the same as is typically applied for product development. In addition, standards must respond to new technological trends such as hybrid insulators (already done in IEC TS 62896), growth of HVDC projects and new field-control products. Indeed, this is being done in cooperation with various D1, B2 and A3 working bodies within CIGRE. Challenges remain, e.g. development of artificial pollution tests for housings made using hydrophobicity transfer materials (HTM) and also artificial ageing tests that better simulate actual service conditions.
Present Structure & Projects
Fig. 4 outlines the present structure of TC 36.
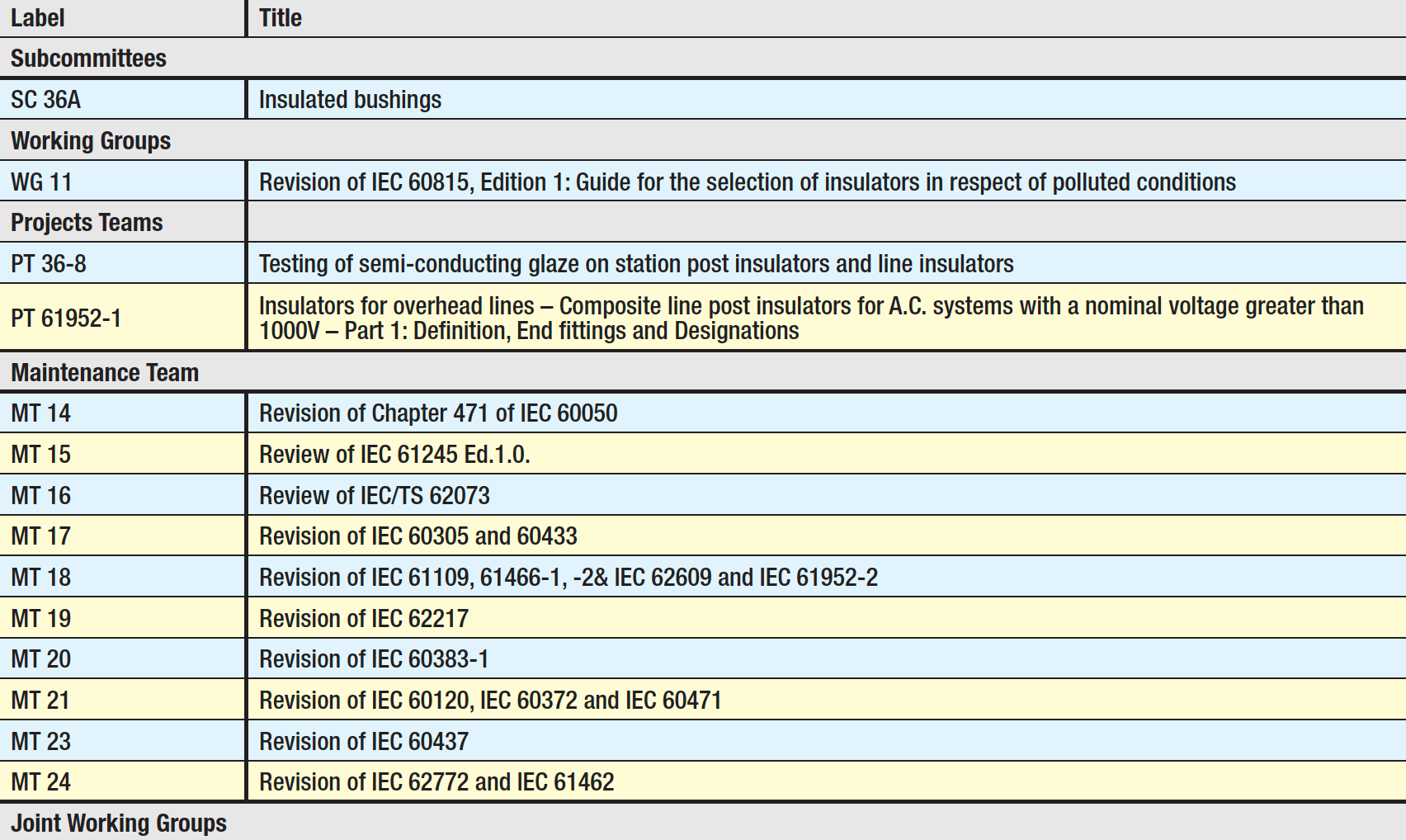
CLICK TO ENLARGE
At the most recent General Meeting of TC 36, in Busan, Korea in late 2018 – an event attended by 24 delegates from 12 countries – the Committee decided on several revisions of existing standards. Such revisions are done by Maintenance Teams (MT), while new work is channeled through Project Teams (PT) or Working Groups (WG). TC 36 has one Sub-Committee (SC), one WG, three PTs, nine MTs and one Joint WG (JWG) with TC 42 ‘Insulation Coordination’. The work program currently has 16 active projects (see Fig. 5).
TC 36 has issued more than 100 publications since 1949, of which 47 are still actively used in the market. MT 18 is a group of experts focused on maintenance of standards for composite overhead line insulators while MT 21 is responsible for coupling systems. Moreover, a new group of experts for composite hollow core and station post insulators (MT 24) was formally established during this latest General Meeting. With all these various activities and number of publications, TC 36 is clearly an active Committee that reflects the high level of industry interest in effective and stable standards for insulators. What can also be seen on the project list is that most composite insulator standards are again under some form of revision (see below for the goals behinds such revisions).
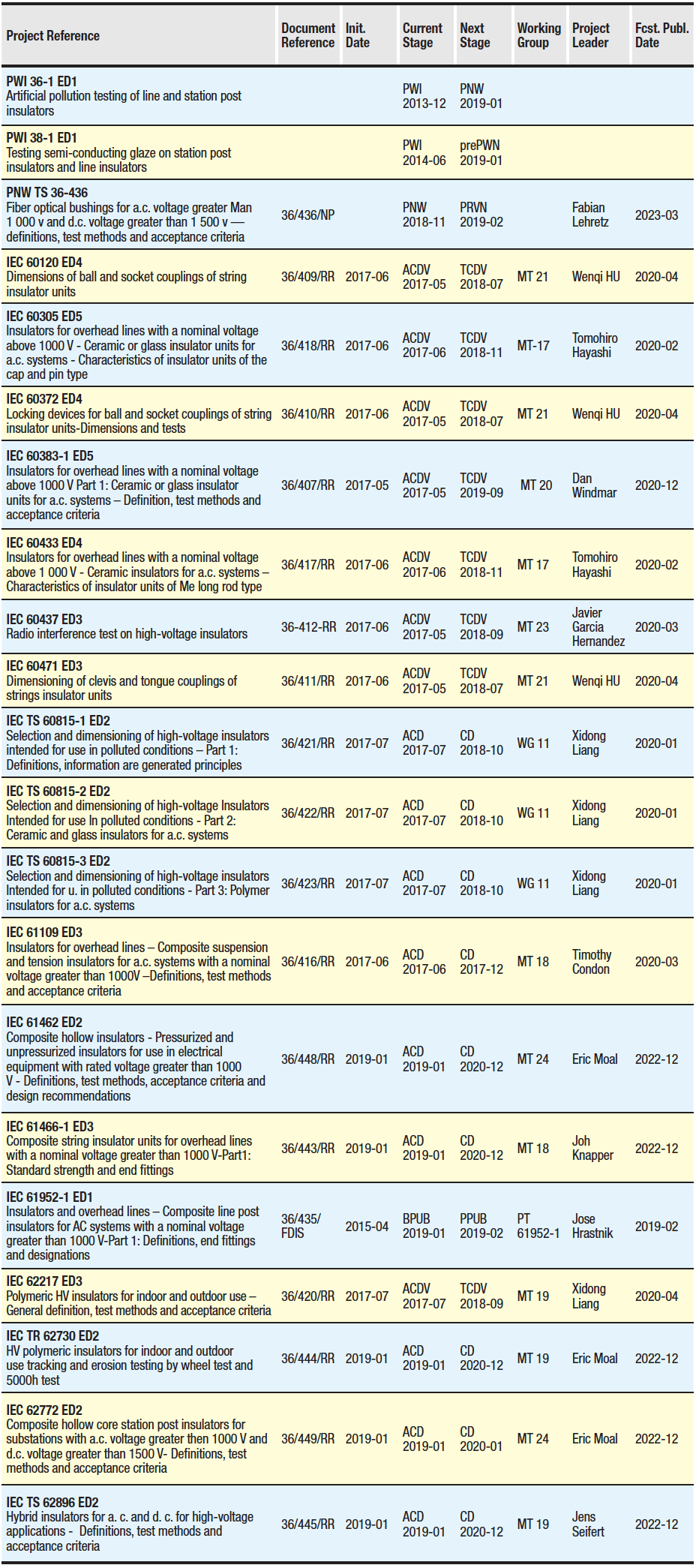
Motivations Behind Revising IEC 61109:Ed. 3.0
• Materials: Need for requirements to be made clearer;
• Interface/Sealing Testing: Improving long-term quality;
• Tracking/Erosion: Making this comprehensive for users;
• A/C and D/C: Is there need for a D/C section?
• Load-time curves & load coordination of components.
Motivations Behind Revising IEC 61952-1:Ed. 1.0
• Creating a standard with dimensions and characteristics;
• Defining typical base and end fittings applied on these products.
This project has since been completed and the standard published.
Motivations Behind Revising IEC 62217:Ed. 3.0
• Better defining and improving the dye penetration test;
• Tests for HTM materials;
• Inclusion of an ageing test (e.g. a modified 5000h test);
• Considering modified (sharper) interface tests.
Motivations Behind Revising IEC 60815 Family of Standards
• Adjusting these documents to better reflect latest knowledge and experience;
• Better defining/characterizing the hydrophobicity transfer (HTM) property;
• Reviewing and adjusting profile factors, especially for DC;
• Improving standards for DC in general.
Motivations Behind Revising IEC 60383-1
IEC 60383-1 offers an interesting example of how improvements can be initiated and revisions triggered in an existing IEC standard. Glass insulator discs have been a proven technology now for well over 50 years. Between 1960 and 2000, there were only four major suppliers of these discs, mostly European based, and these served the world market. Materials and designs were more or less standardized in terms of ‘best practice’ and this included shapes of assembly interfaces, cementing processes and material/glass composition. By 2014, however, the number of suppliers of glass insulators had jumped to more than 20 and many of these focused on cost-driven production. More manufacturers entered the market with different design approaches (e.g. in glass formulation, cement and assembly technology) and production also shifted more and more to developing countries, mostly in Asia. Today, there is evidence of a large scatter in the quality of glass discs due to non-standardized materials and production methods combined with uncertain quality control and process stability. The inability of the current IEC 60383:1993 standard to detect such deficiencies was expressed in two papers presented during the meeting of SC D1 at the CIGRE General Sessions in 2014 and 2016.
At the moment, there is no IEC standard available to detect possible variance in quality of glass disc insulators. Since 1992, all new standards (e.g. design tests) were developed only for composite insulators in order to implement test methods that could identify and reject inferior designs and materials. By contrast, IEC 60383:1993 has not been modified in any way since inception more than 25 years ago, when there was high correlation between this standard and product quality in the marketplace. In this regard, it could be said that the market situation changed but the standard did not. As recognition of this problem grew among utility users worldwide, the Swedish National Committee proposed revising IEC 60383 during the last plenary meeting in 2016 and this project has now been allocated to MT 20. This demonstrates that standards need to be adjusted periodically to reflect changes in the marketplace.
Fortunately, new professionals entering this field actively support CIGRE/IEC and are in balance with experienced experts. This is important to secure the necessary human resources in the future while also conserving industry knowledge. Interest in this work is high, as reflected by the level of industry participation in active projects. In the future, IEC standards will be organized with the following generalized document structure, e.g. for IEC 61952:
Main Part: IEC 61952: Terms, definitions, test methods and acceptance criteria
Part 1 – IEC 61952-1: Dimensions & characteristics
Part 2 – IEC 61952-2: Application guide
The application guide will give further product and application information, e.g. in the case of braced line posts to better understand complex topics such as load-time curves and load co-ordination.
Conclusions
Tests documented in IEC standards must satisfy all the following requirements: repeatability, reproducibility, representativeness and cost efficiency. Standardization is a process of continuous improvement and ‘learning’. Assistance by CIGRE is essential in order to base IEC standards on sound scientific principles as well as consolidated industry knowledge. Reaction time must be improved, especially for new and emerging technologies, to shorten the time to standard as well as the time to market. DC technology must be better supported from both the product and test standard sides. IEC standards must, of course, follow innovation but in some cases standards have to react to and also adapt to market as well as product quality changes