Transmission and distribution companies are a significant source of different solid waste materials including porcelain bushings and insulators, surge arresters, transformers, etc. However, waste management policies for such types of scrap components have only started to evolve and landfill has often been the dominant way for disposal.
This edited past contribution to INMR by Dr. Robert Sekula, then at ABB Corporate Research in Poland, proposed recycling options for different insulation products and discussed the results of laboratory experiments carried out to assess these options.
Ceramic and thermoset materials are used widely in the electrical industry due to superior dielectric properties as well as mechanical strength that together make them ideal for insulators, bushings, instrument transformers and other components. An appropriate waste management policy must therefore ideally be available in relation to reutilization/recycling of scrapped components. Legislation in Poland, for example, is based on European Directives and has indicated that waste management development should be concentrated on wastes that meet the following requirements:
• can be used as raw materials for a production process;
• can partially or completely replace raw material or fuel being used so far in a production process;
• can be used to improve a quality and an efficiency of a production process;
• can be used to decrease a hazardous effect of the process on the environment;
• can be a source of valuable materials;
• after processing can become a valuable product.
[inline_ad_1]
Three streams of the wastes were investigated in this particular past research: scrap plastics (thermosets), scrap ceramic insulators and scrap silicone-housed arresters. Although large volumes of these items are used on overhead lines and at substations, there is often no rational policy governing their disposal and landfill has been the dominant management policy for such wastes. It should be pointed out, however, that the various products used in the electrical industry usually have different metals embedded inside cured thermosets and silicones. Their recovery could therefore prove a valuable recycling operation.
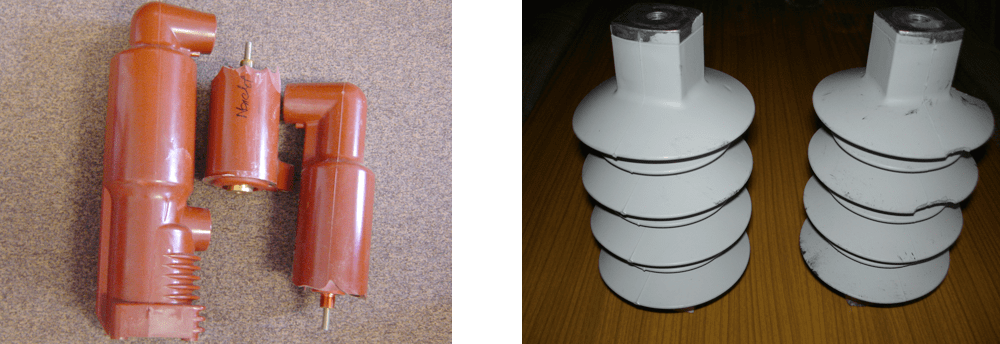
CLICK TO ENLARGE
Scrap Thermosets
Given the increasing volumes of various scrap materials and limited landfill space for their disposal, more sophisticated recycling oriented technologies will need to be promoted. The following options have been considered in the past for utilization of thermoset wastes:
• application as construction materials;
• energy recovery;
• degradation
Use of cured epoxy based scrap in concrete or asphalt construction materials has been considered many times and seems a simple way of utilization. But given that the amounts of such materials are relatively small and waste streams are geographically distributed, there is relatively low economic benefit in such solution. It should be noted that epoxy scrap is widely used in electrical equipment and therefore various internal parts made of metals (e.g. cores, windings, etc.) are embedded in the epoxy. This implies the need to apply some pre-treatment process to the scrap, such as removal of metallic parts followed by crushing. Considering the high energy content of thermosets, (LHV from 10 to 20 MJ/kg depending on filler content), energy recovery by combustion offers a valuable option. However large amounts of inorganic matter in the form of filler are generated in such treatment and must be handled in some economical way. From the air pollution point of view, combustion of thermosets is seen as relatively safe given a properly defined process whereby hazardous emissions can be limited. Another utilization option is degradation and this refers to reduction of plastics to lower molecular weight materials through such processes as photo-degradation, chemical degradation and biodegradation. Pyrolysis is a specific form of chemical degradation and the research presented below has focused on that method of thermoset scrap utilization.
Scrap Ceramic Insulators
So far, the problem of utilization of scrap porcelain insulators has not been greatly emphasized. Scrap porcelain insulators can be ground up for use in road aggregate, ice melt and outdoor tiling. Sometimes, used porcelain insulators can be re-utilized by the manufacturer as raw material for manufacturing new insulators. But, more typically, these have also been stored on site at substations, often without any disposal solution or they have simply been buried.
It is clear that development of a more versatile technology and implementation of an efficient waste management strategy for large numbers of porcelain insulators has become a necessity. This was the reason for investigation of a new approach to resolve this problem.
[inline_ad_2]
Proposed Recycling Solution for Thermosets
Any recycling option for thermoset-based components such as epoxies and silicones has to take account of the fact that valuable components made of metals such as copper and aluminum are often embedded in the insulation material. Therefore any proposed methodology must result in a high quality of recycled parts. Given both ecological and financial aspects, pyrolysis was selected as a utilization process, allowing thermal degradation in an oxygen-free environment and resulting in number of end products, i.e.:
• pyrolytic gas;
• liquid products;
• solids (char, mineral fillers, metals).
Epoxy Scrap Products
The pyrolysis process was carried out using an experimental arrangement as shown in Fig. 2. The pyrolytic reactor comprised of electric heaters and thermocouples were the main parts of the system. Design of the reactor allowed regulation of the temperature inside across a wide range, achieved using a type K thermocouple connected to the reactor’s temperature regulation system.
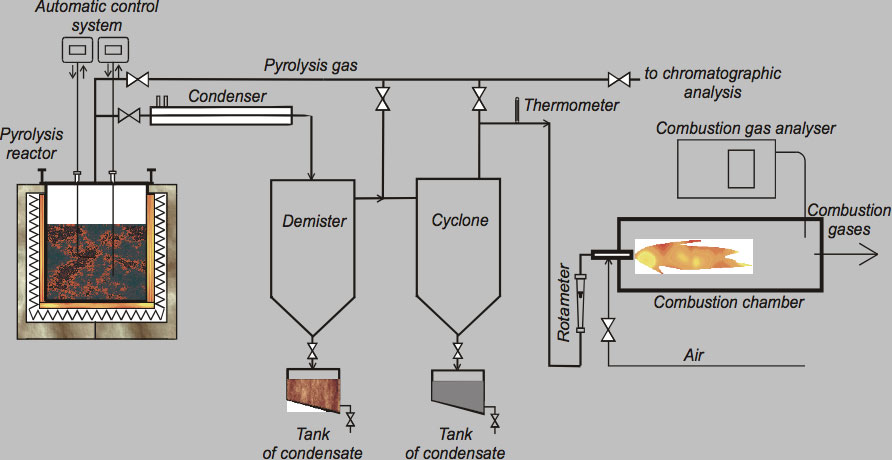
CLICK TO ENLARGE
Condenser, demister and cyclone are the main components of the purification system for the pyrolysis gas, which was burned inside a combustion chamber equipped with a Bunsen burner. Liquid products are separated by condensation and collected in the demister and cyclone. Minimal time for proper pyrolysis was about three hours for one feed of processed material and maximum time was about 5 hours. Low temperature pyrolysis was conducted at 450°C and a high temperature process performed at 750°C and 850°C. In total, three different sets of experiments were performed to find optimal process parameters that assure high decomposition of organic material (i.e. low carbon content in solid residues) as well as good quality of the recycled metallic parts. Table 1 presents sample results of such well-defined pyrolytic recycling showing the gaseous end products while Table 2 covers the solid end products.
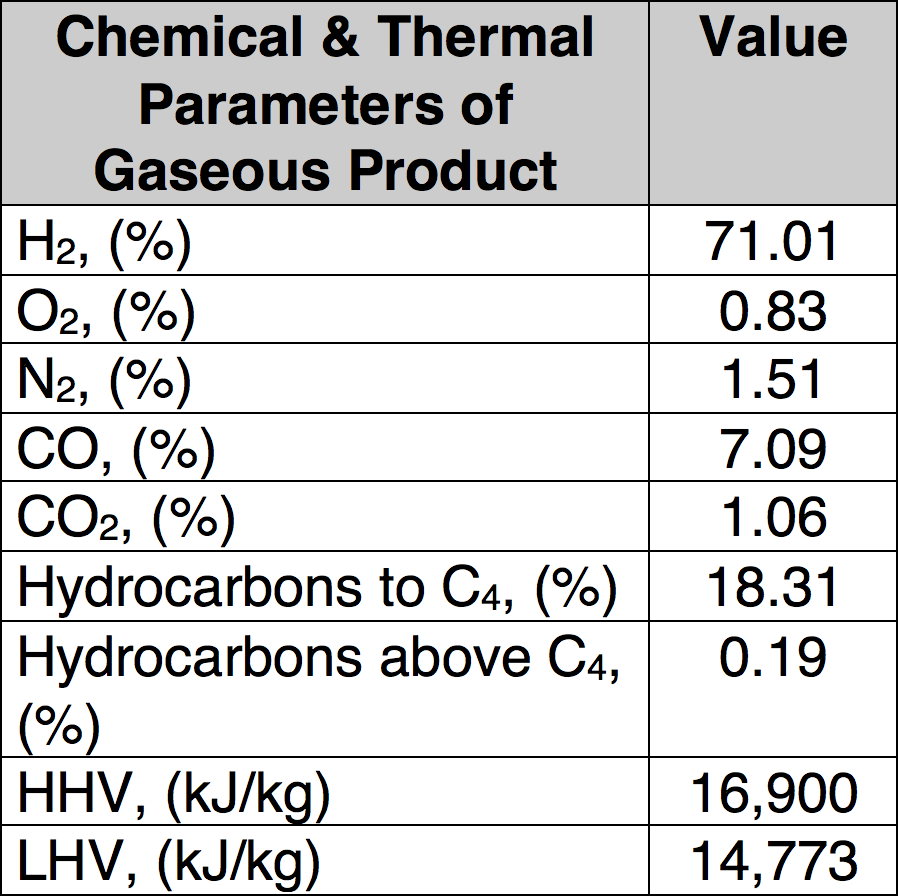
CLICK TO ENLARGE
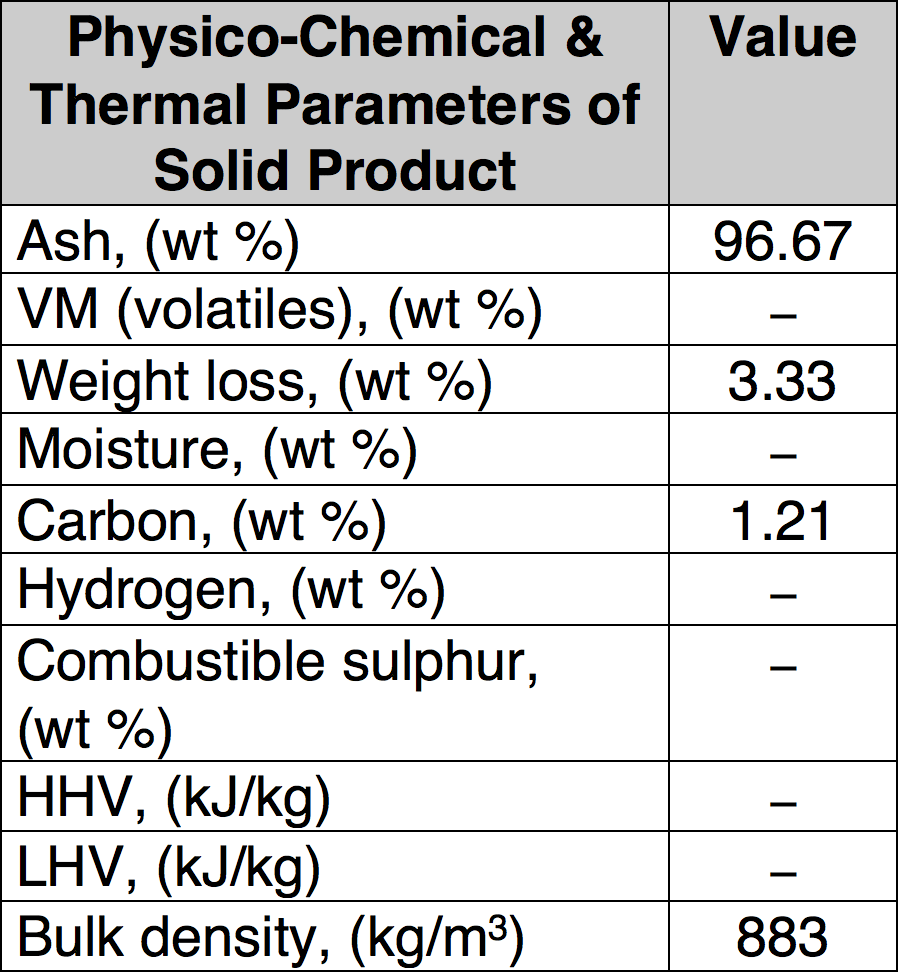
CLICK TO ENLARGE
Based on these experiments, it was found that pyrolysis can indeed be used for thermal utilization of resin wastes with simultaneous recycling of metallic components. Pyrolysis end products, whether gas and oils, can also be utilized as fuel in the next stage in this technology of waste utilization with potential for their recovery as a source of energy.
Scrap Surge Arresters
During past decades, silicone-housed surge arresters have found broad application across medium as well as high voltage systems. Recycling investigations have been performed for insulation made with liquid silicone rubber (LSR) but the number of efficient decomposition methods is still fairly limited and mostly combustion (i.e. incineration) is used. But this process is characterized by temperatures of over 900°C and its application in recycling surge arresters is not accepted given degradation of the zinc oxide varistors and emission of heavy metals. Nonetheless, it should be noted that, from an energy standpoint, combustion of silicone rubber results in high release of heat due to relatively high heat of combustion, reaching 17,000 kJ/kg (whereas heat of combustion of hard coal is 25,000-30,000 kJ/kg). Given the above, the only rationale option for thermal degradation of silicone is based on pyrolysis and therefore the same experimental set-up used for epoxy wastes was applied. Again, different temperature scenarios were used to optimize process parameters with the aim of recycling good quality internal parts (in this case mostly the zinc oxide varistors). These experiments resulted in development of an efficient procedure and Fig. 3 shows samples of post-process products.
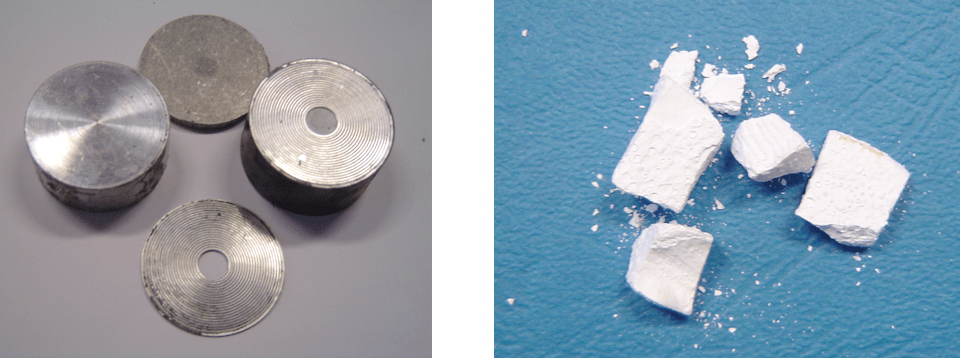
CLICK TO ENLARGE
Solution for Ceramic Insulators
As mentioned, development of interesting techniques for utilization of scrap ceramic insulators has been relatively limited. In general, porcelain is not considered dangerous to the environment and landfilling is used broadly. However due to limited space for this option and growing fees, it has been decided to explore novel utilization methods. These investigations started with detailed analysis of the composition of the porcelain used as electrical insulation, as presented in Table 3.
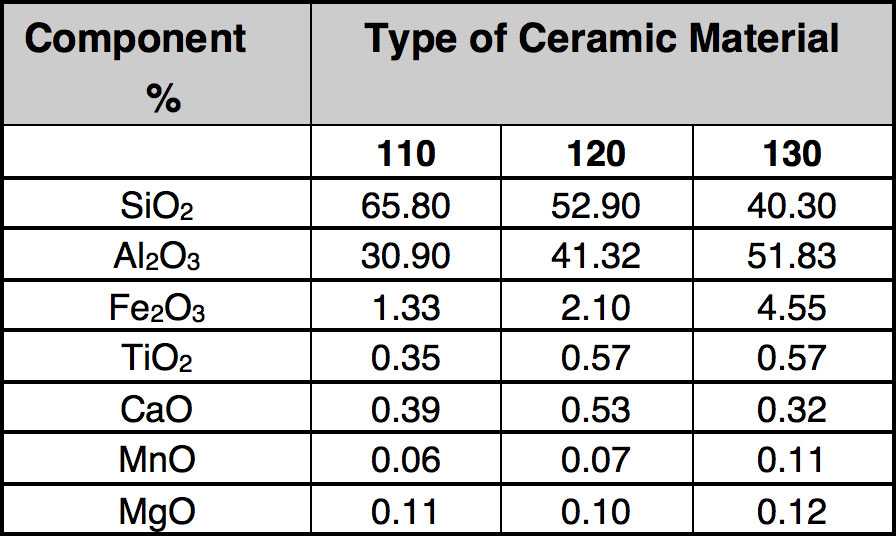
CLICK TO ENLARGE
Analyzing the data, it is clear that reported oxides are mainly in the Portland cement (see Table 4). This was then the driving factor for development of a potential utilization of scrap insulators in rotary cement kilns. The process is based on grinding the porcelain insulators and injecting the powder (preferably in a mixture with pulverized coal) into the flame inside the cement rotary kiln (i.e. at the hot end). There has been a suggestion that this can promote catalytic reactions on surfaces of particles leading to reduction of NO to atmospheric nitrogen. As mentioned, insulator composition is close to that of cement raw material and therefore addition of milled insulators to the kiln should be acceptable due to the increase in yield.
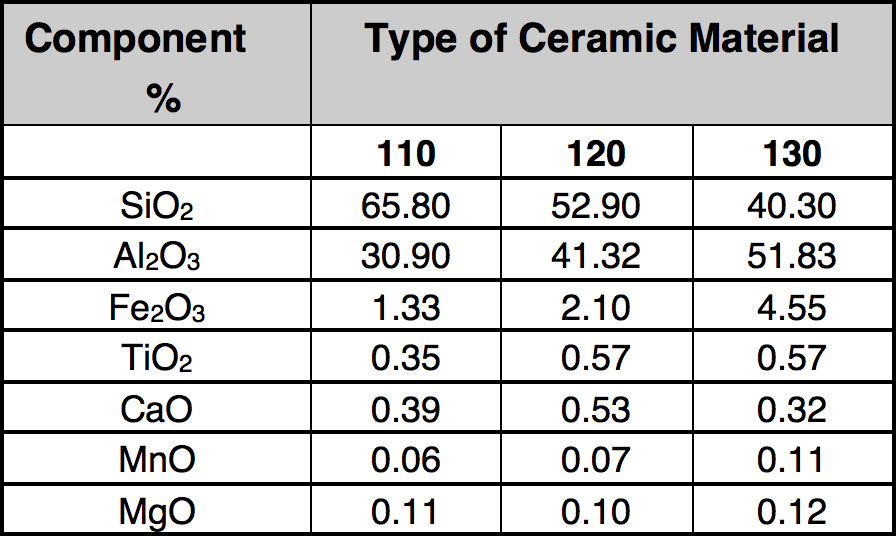
CLICK TO ENLARGE
To verify this hypothesis, laboratory tests were performed with the goal of checking the influence of introducing ceramic powder, obtained by grinding scrap ceramic insulators, into the flame. Three types of ceramic material (C110, C120, C130) were tested. To assure high surface area of the powder, ceramic insulators were milled to grain size below 200 m. A laboratory furnace was the main part of the set-up (see Fig. 4) with a 1.3 m long combustion chamber having inside diameter of 0.25 m and equipped with an oil burner of 7 kW thermal capacity. The furnace had 10 sampling ports along the combustion chamber.
[inline_ad_3]
Diesel oil was mixed with ceramic powder in a mixing tank and supplied to the burner. Three grams of ceramic powder per liter of fuel was added and a TESTO 350 analyzer and water-cooled probe were used to measure flue gas concentration. Temperature of the flame and flue gases was measured with a Type S thermocouple and all measurements were conducted along the furnace axis. Due to very low NO2 concentrations (in the range of 1 to 10 mg/m3), studies focused on nitric oxide, NO. To increase the amount of nitrogen oxides generated in the process, up to 10% by weight of pyridine (C5H5N) was added to the diesel oil. This allowed obtaining NO concentration in flue gases above 2000 mg/m3.
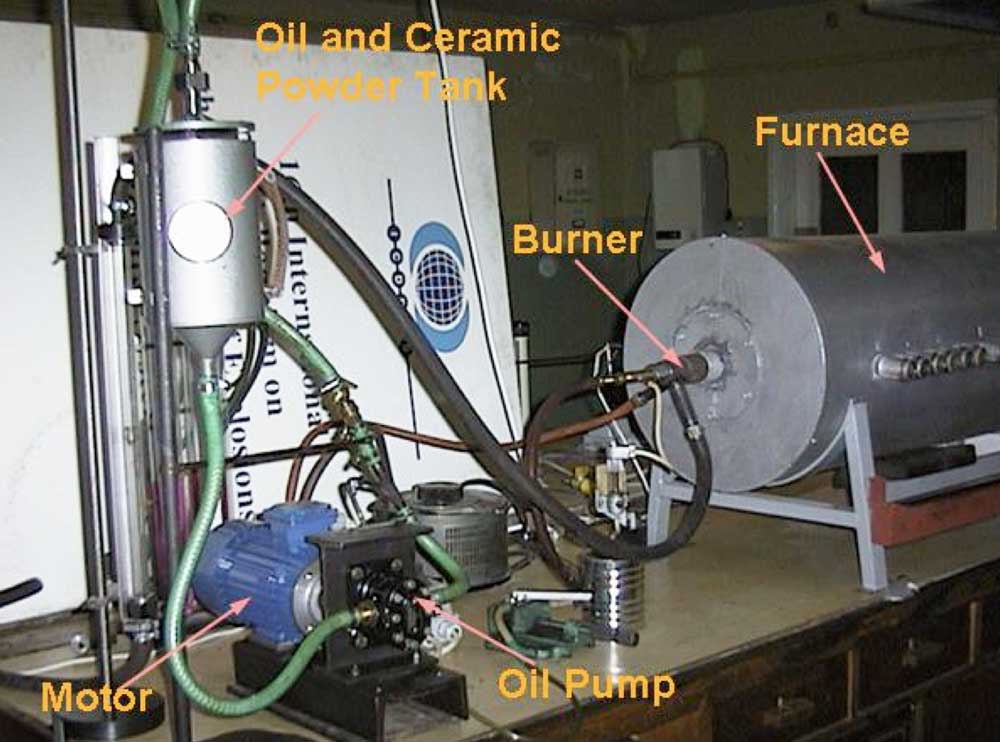
CLICK TO ENLARGE
The experiments on oil combustion allowed observing the influence of injecting ceramic powder on the concentration of nitrogen oxides, mainly in the fuel. To check the effect of the process on thermal nitrogen oxides, additional tests were later carried out in the same equipment but using natural gas as fuel. In this case, the oil burner was removed and a natural gas burner of 6 kW thermal capacity was installed along with the powder injection system.
Figs. 5 and 6 present sample results of these experiments. All NO concentrations were recalculated for 3% oxygen level to allow clearer comparison of results for combustion tests with and without powder injection. It can be seen that introduction of ceramic powder to the flame affects NO concentration and a significant reduction in nitric oxide was achieved, in many cases by more than 50%. The positive effect in regard to lowering NO emissions was observed for both fuels – oil and natural gas. This means that injection of the ceramic powder to the flame zone influences both fuel and thermal nitrogen oxides.
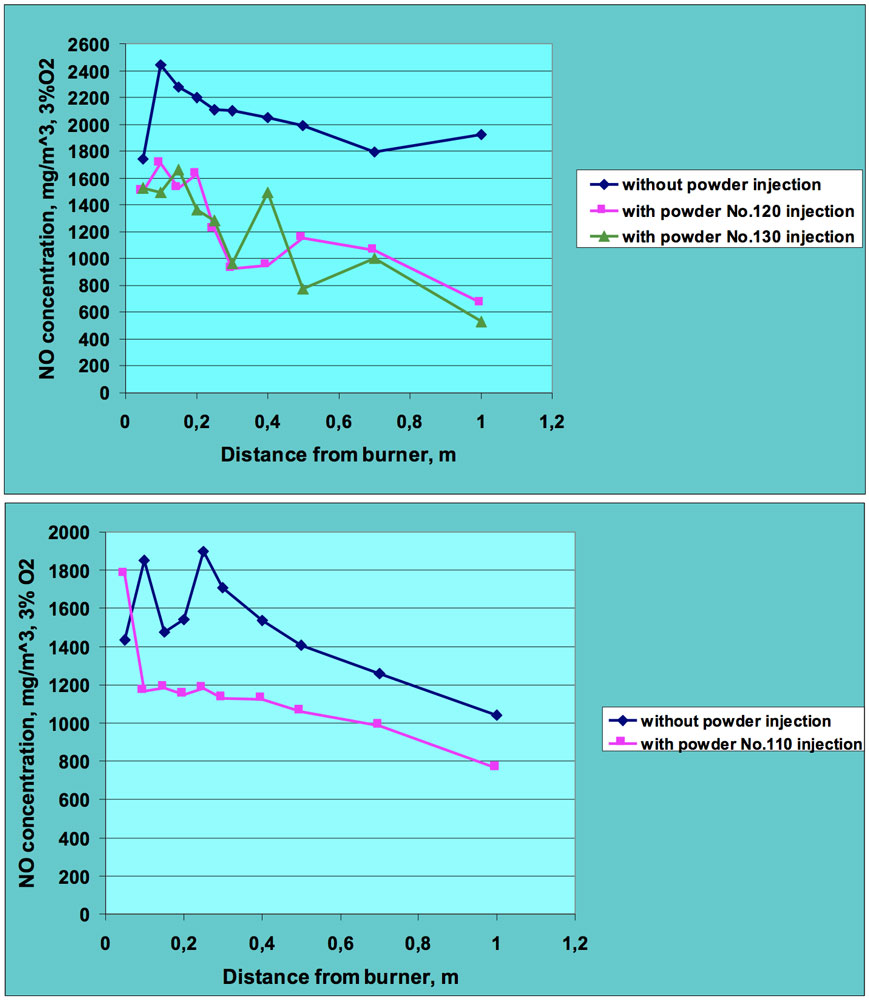
CLICK TO ENLARGE
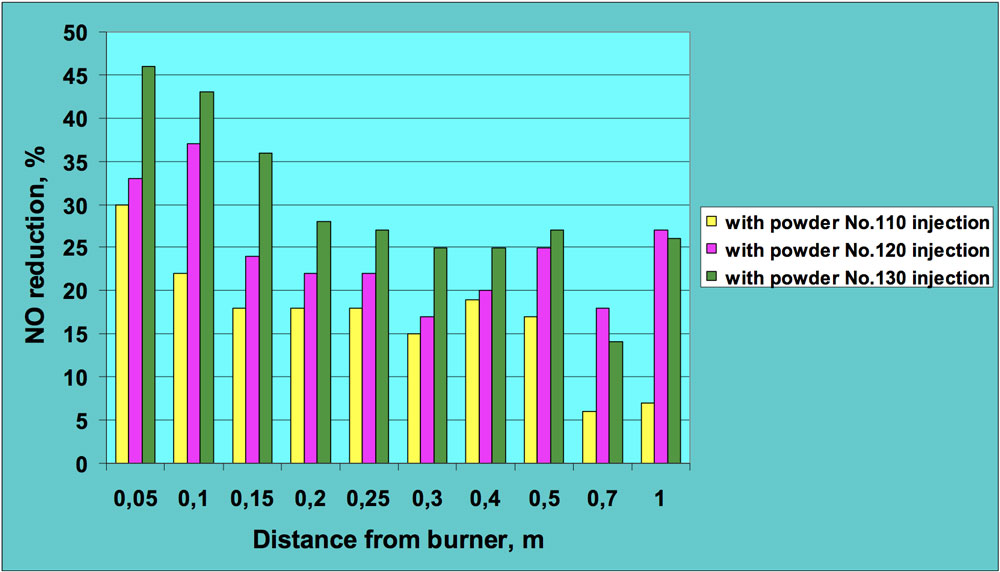
CLICK TO ENLARGE
The explanation for this phenomenon is based on the assumption that there is some catalytic effect of ceramic powder components involved. This may be the same effect observed during research by DeSoete where he pointed out that NO can be destroyed in the presence of a reducing agent (e.g. CO) on both cenospheres and even on the wall of an empty quartz reactor. DeSoete derived rate expressions for destruction of NO and HCN on fly ash, gas phase formed soot and cenospheric soot. In all cases, the nitrogenous species was efficiently destroyed. Components found in ceramic insulators are almost the same as found in fly ash and therefore may also promote NO reduction. However, the influence of metal oxides concentration in the ceramic powder on reaction kinetics of NO and CO on the powder surface was not well defined at time of this research. Therefore, this process required further investigations, including kinetics of in-flame reactions in the presence of solid porcelain particles with differing amounts of powder being added.
[inline_ad_4]
Conclusions
Laboratory investigations confirm that available (now patented) technologies exist for recycling different types of insulation components. But successful commercialization of these solutions requires waste disposal organizations to implement appropriate policies for efficient collection of these scrap components, supported by local governments.
References:
1. Polish Environment Agency.1998. Current Environmental Rules. Koszalin
2.Bledzki A.1997: Recycling of Polymeric
Materials, WNT, Warsaw (in Polish).
3. Pickering S.J., M. Benson. 1991: “The
Recycling of Thermosetting Plastics”,
Plastic Recycling Meeting, London.
4. Reason , J. 1990. Overhead Distribution
Electrical World, 204.
5. Czerski, L.1967. Chemistry for Technicians,
Warszawa (in Polish).
6. DeSoete, G.G.1980.Heterogeneous Nitric
Oxide Reduction on Flame Borne Solids Particles. Proceedings of Sixth Members Conference, IFRF, Noordwijkerhout.