Three proven insulator technologies are deployed in delivery of electrical power on overhead lines. Porcelain and glass insulators have been used since the start and have had a solid record of reliability and longevity. However, their performance has not always been satisfactory, notably under high pollution or in areas with high rates of vandalism. In both cases, problems have emerged with the need for costly maintenance involving cleaning, coating or replacement.
Composite insulators were developed over 50 years ago largely due to these deficiencies and introduced on an industrial scale during the 1980s and 90s. While there has been a learning curve as suppliers developed successive generations of design based on growing service experience, the technology has basically achieved maturity. In fact, it is now generally considered equivalent and fully interchangeable with porcelain and glass. As a result, one can often find a mix of all three technologies applied on power systems, often on the same line and sometimes even on a single tower.
Since insulator technologies share common standards, some users have come to believe that ageing and maintenance issues should also be the same. But this is not always the case. As reviewed in this edited past contribution to INMR, electrical, mechanical and environmental service stresses can result in significant differences in types and rates of failure, often relating to different materials, designs, manufacturing methods and handling practices.
Manufacturing Processes
High strength, electrical grade porcelain for line insulators is most often manufactured using the classical wet process. Raw materials (mainly clay, quartz, feldspar and corundum), which can vary in quality, purity and consistency, are mixed in water to facilitate blending. The water is then progressively removed in several steps up to firing the porcelain in kilns where the temperature cycle is carefully controlled. A glaze is applied prior to firing, forming a smooth surface and at the same time improving mechanical strength of the porcelain body. Finally, hardware is attached, typically using Portland cement. While all electrical porcelain might appear uniform, it is important to note that at the microscopic level it remains heterogeneous, containing crystals of various sizes and chemistries having different pores and grain boundaries.
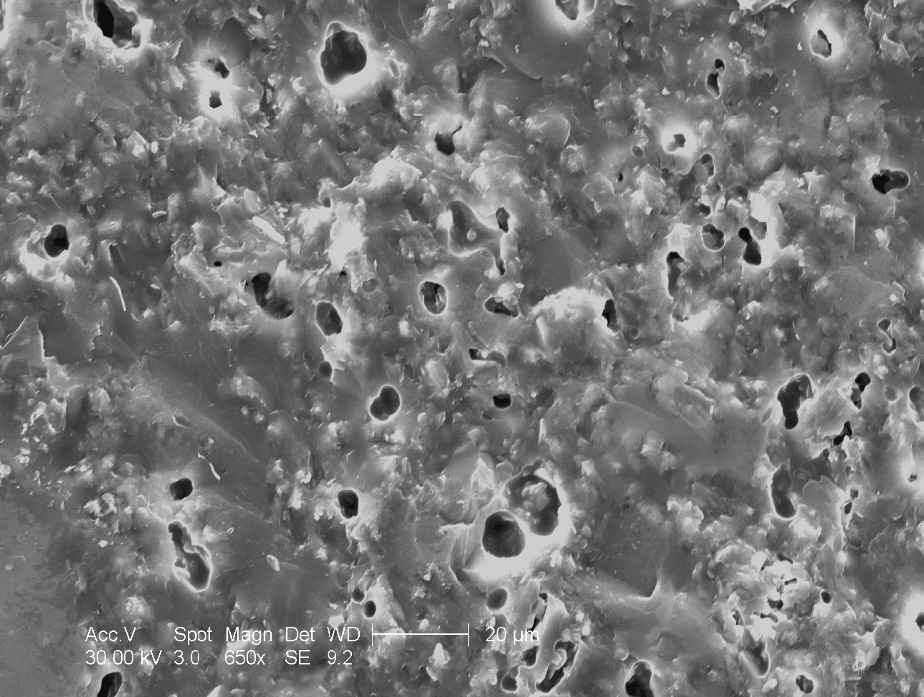
The raw materials for glass insulators include soda ash, feldspar and cullet (pieces of glass from earlier production cycles) as well as minor amounts of other ingredients. These are crushed to form powder and melted in a furnace. The molten glass drops into a mold and is pressed into the desired profile before being subjected to toughening by means of rapid, controlled cooling of the still hot shell. Toughening significantly increases mechanical strength, making the glass shell suitable for high strength cap & pin strings.
Composite insulators consist of a central fiberglass core, a polymeric housing material and metal end fittings. The core provides the mechanical and electrical strength and is made by pultrusion of unidirectional glass fibers bonded in an epoxy resin. Since the core can degrade in the presence of moisture and electric stress, it has to be protected. This is a main function of the external polymeric housing and seals. Housings are expected to resist weathering as well as damage from electrical activity such as corona and surface discharges that can occur under wet, contaminated conditions. The method of fixing the housing over the core differs among manufacturers. Some mold the complete housing over the rod in sections while others use a combination of a sheath extruded over the core and individually molded sheds that are chemically bonded to the sheath. Seals at the hardware junctions prevent moisture ingress, although some manufacturers mold the housing directly over the hardware at the critical triple point to perform this function.
Modes of Failure
Each failure mode has specific consequences on service performance of an insulator and the string that contains any failed units. In some cases, failure will result in complete loss of service, as in separation of the cap from the pin or break of the fiberglass core rod – both resulting in dropped conductors. In other cases, less serious failures might occur such as partial damage to discs or housings or shattering of the glass shell, which most often has no immediate consequence on safety and integrity of a string.
When reviewing failure modes of different insulator technologies, it is important to qualify these based on comparing the risks and results of such failures. Below is a summary of principal modes of failure:
1. Pin or Cap Corrosion
Problems of pin or cap corrosion occur in places with severe contamination and are basically independent of dielectric bulk material, whether porcelain or glass. In the case of composite insulators, the polymeric long rod between points of different potential limits leakage current to such small values that hardware corrosion is not a serious risk. Use of newer designs with sacrificial zinc sleeves for improved corrosion protection is a proven way to avoid onset of this problem but of course has no impact on ageing of the existing population of discs from earlier generations.
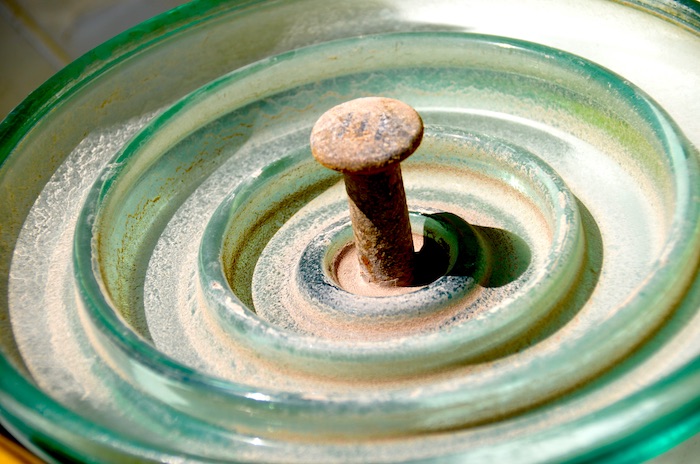
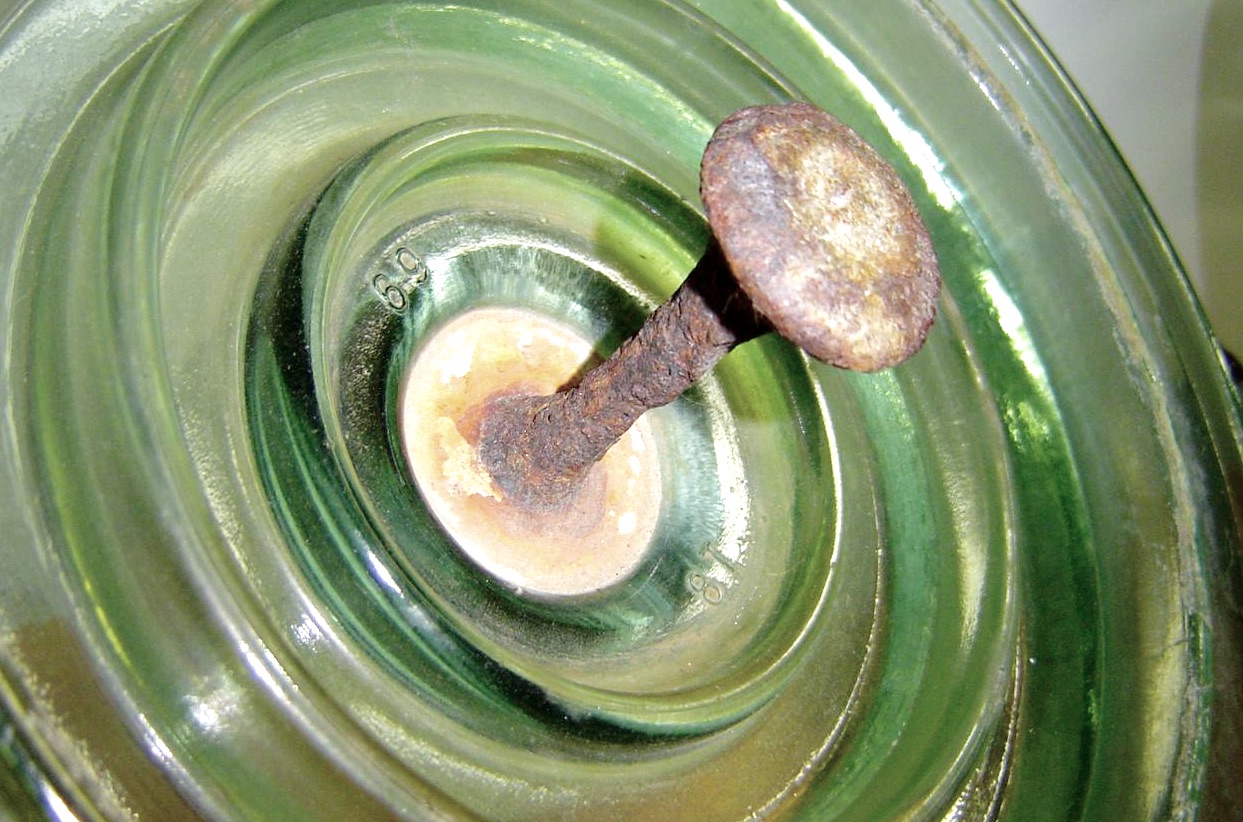
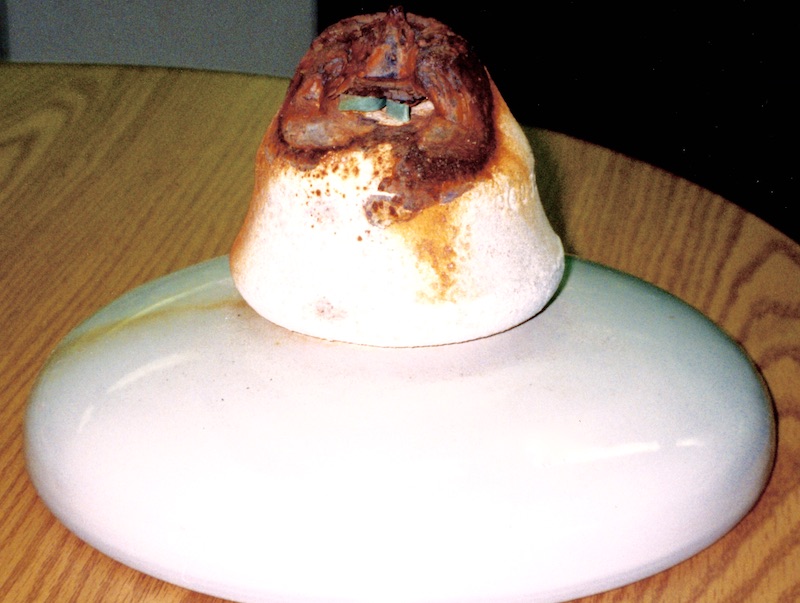
2. External Flashover
This mode of failure occurs externally to the insulator and results in temporary loss of insulation strength. Occurrence depends mainly on pollution conditions and lightning activity as related to the key design parameters of the string (i.e. leakage and dry arc distances) as well as the system’s insulation coordination. For any given leakage distance, flashover is a more common problem for porcelain and glass insulators due to their relatively easy wettability compared to composite insulators that utilize low surface energy housings that resist water filming.
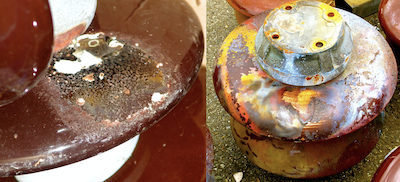
Comparing porcelain and glass insulators of any given leakage distance, shed profile and string design parameters, there have been no significant differences in reported rates of external flashover failure. But the power arc current typically causes the glaze in porcelain units in contact with the arc to melt, leaving behind a rough surface that is more susceptible to pollution accumulation and eventual failure. Composite insulators, by contrast, are more resistant to power arcs with only superficial burning and/or ablation of the housing observed. While some melting of hardware may occur, there is generally no internal damage.
3. Internal Puncture
This failure mode is seen in porcelain insulators and relates primarily to problems with raw materials and production processes. Some manufacturers have inferior quality control methods and this impacts not only macroscopic properties but also microstructure of the porcelain body itself. For example, the microstructure of insulators that have passed all relevant tests in their new condition can feature presence of pores (voids) and numerous grain boundaries between crystals, as common to all porcelain. But sometimes there are micro-cracks that can grow under the multiple stresses generated by service conditions and eventually lead to electrical breakdown through the bulk dielectric. Internal punctures of porcelain insulators are detectable only through close-up inspection of the line using measuring instruments.
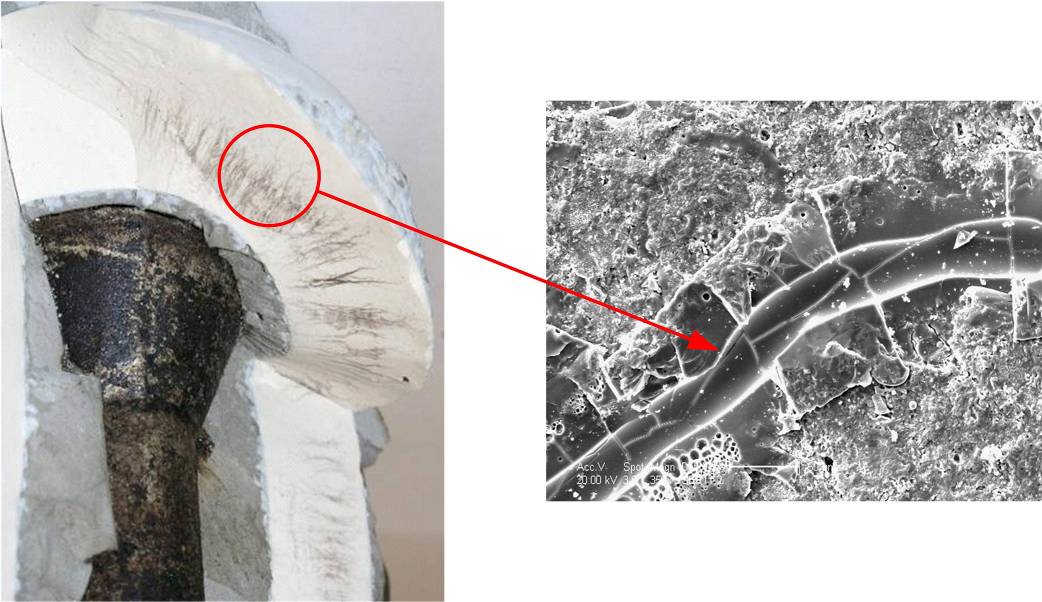
Reliable statistics for this type of failure are not available on a global basis. But experience at many power supply companies shows that this problem, while rare in new insulators, is more common on older lines, especially if the porcelain insulators were not of high quality to start.
Composite insulators are not normally at risk of puncture since the electrodes of different potential are separated by a long fiberglass rod. However, punctures in the form of holes in the housing have been reported in some applications and these provide an access for moisture into the rod. Tracking along the rod under the housing material has then been known to occur, resulting in permanent loss of electrical strength – just as in a puncture. If the internal tracking is considerable, the insulator will not be able to support the electrical stress and the rod will essentially become carbonized along its length. This mode of failure is sometimes referred to a ‘flashunder’ and can also result from damage to the housing from mishandling during transport or installation.
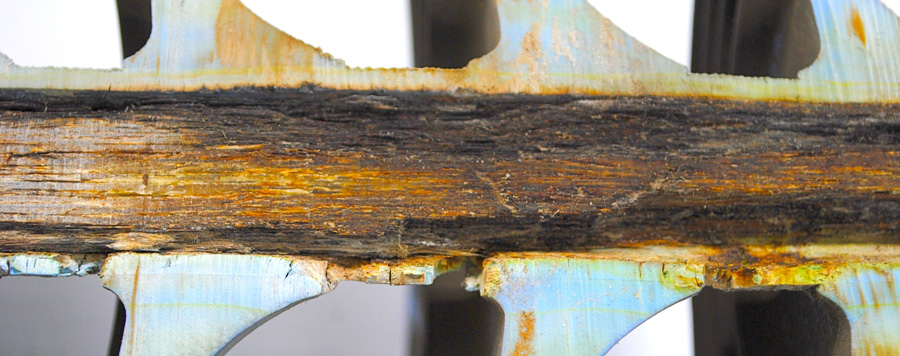
Puncture of toughened glass insulators is seldom seen in service. The increased strength from the toughening process derives from opposing balanced tensile stresses induced inside the glass body and compressive stresses throughout the outer surface. As a result, a glass shell can either shatter completely or remain intact. Moreover, since glass is essentially a cooled liquid, there are no interfaces within the dielectric. The toughened shell is immune from hidden internal micro-cracks or punctures that would cause a disc to shatter. Therefore, it always breaks in a predictable pattern. While it is feasible to puncture the dielectric in the laboratory with high electric stresses, such stresses are not common in service. Even when the shell is broken, the external air insulation of the stub breaks down before the electric stress can rise to higher values, thus eliminating dielectric puncture.
Even in case of flashover of the string, the arc will be external to the stub since its internal impedance is significantly higher than that of the air gap between cap and pin electrodes.
4. Mechanical Separation
Such a mode of failure is comparatively rare (but not unheard of) in the case of toughened glass insulators. Operating data reported by utilities suggest that failure rate is extremely low – on the order of only about 1 in 10 million. This problem is generally associated with exceptional events such as repeated power arcs that melt the fittings or uncontrolled vibration of phase conductors propagating to the insulator strings and leading to fatigue breakage of the pin.
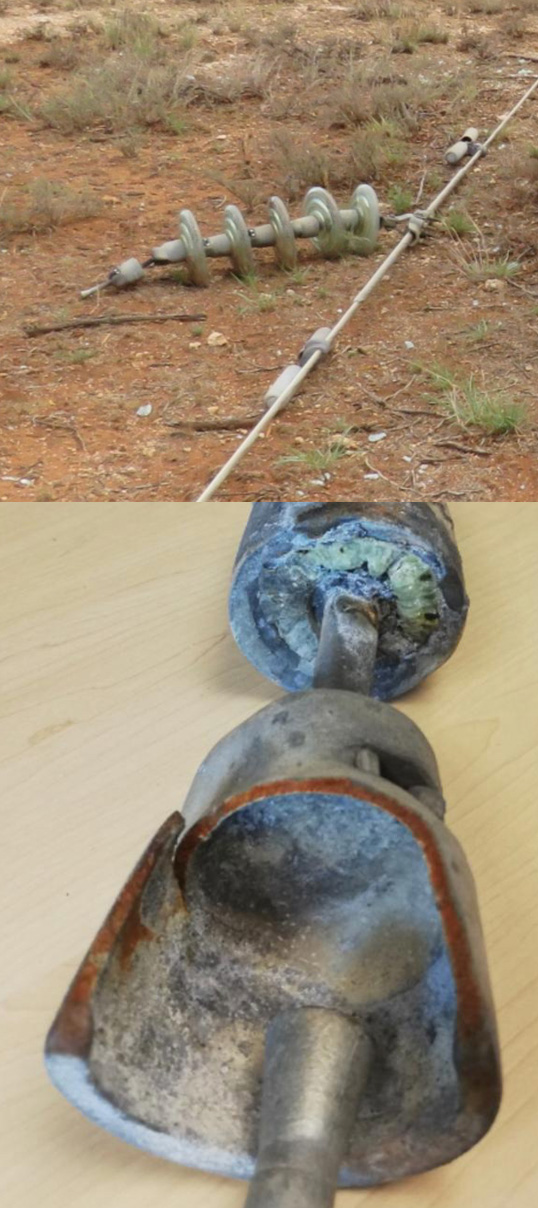
By contrast, service experience indicates that separation of strings of porcelain insulators – sometimes referred to as ‘de-capping’ – is far more common. This is largely associated with time-related ageing, leading to cracks and puncture of porcelain shells under continuous electrical and thermo-mechanical stresses. The separation of cap and pin occurs when the string flashes over (e.g. triggered by voltage surges from lightning or switching events or by pollution). The power follow current can produce such large electromechanical forces in the punctured units as to cause the porcelain to separate from the cap and result in line drop.
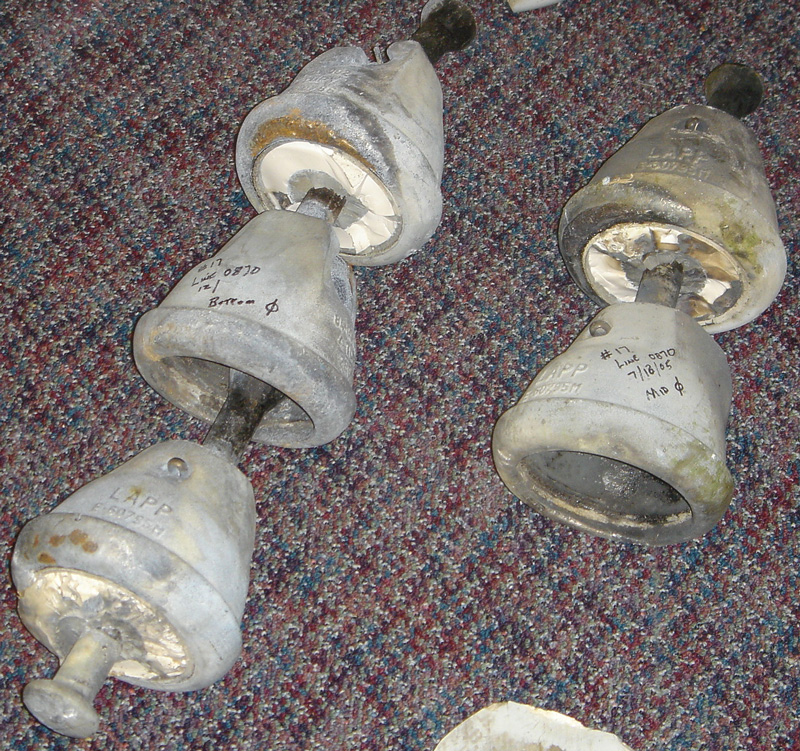
Brittle fracture of composite insulators leads to mechanical separation of the fiberglass rod, also causing the conductor to drop. This failure mode was initially reported in some first generation insulators manufactured in the 1970s and attributed to attack on the rod by nitric acid produced by corona in a moist environment. The exact source of the acid later became a topic of research and debate. While there is sufficient proof that electrical stress is not required for brittle fracture to occur, it can nevertheless accelerate the process. There is also evidence that certain types of chemicals used in manufacturing fiberglass can themselves produce acids, even without moisture from the outside. It is commonly accepted that effective sealing of the rod from the elements and use of corrosion resistant rods is the most effective way to minimize this type of failure. It is likely that brittle fracture is due to a variety of factors and cannot always be explained by one mechanism alone.
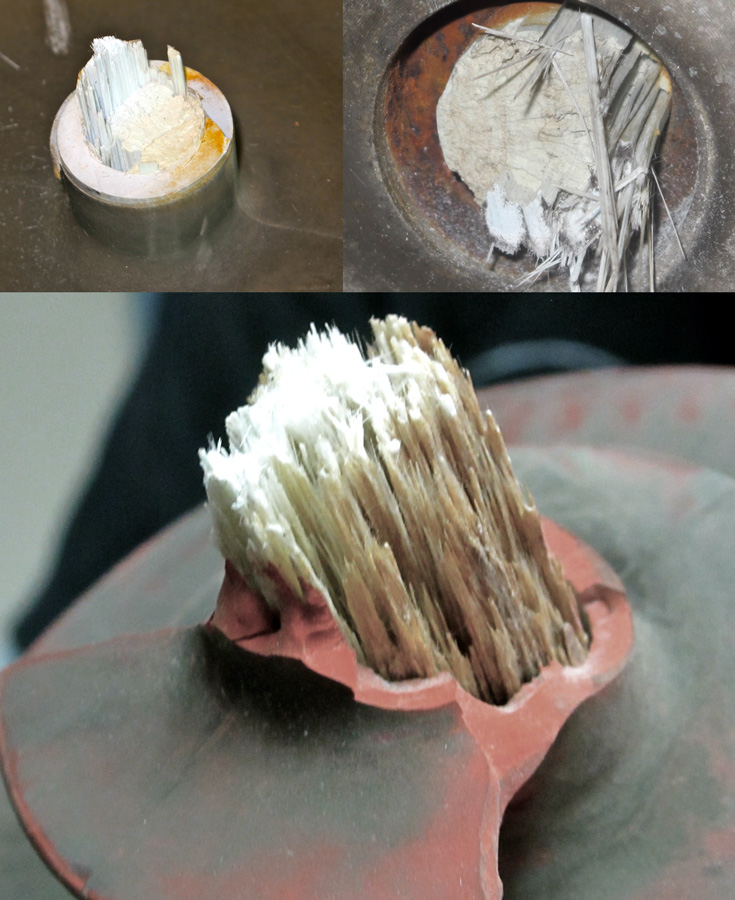
5. Partial Breakage/Damage in Dielectric Material
This type of failure is evident in porcelain insulators and typically caused by vandalism or poor handling during storage and installation. Unlike toughened glass, only a section of the porcelain disc is broken and any additional internal cracking cannot be easily ascertained.
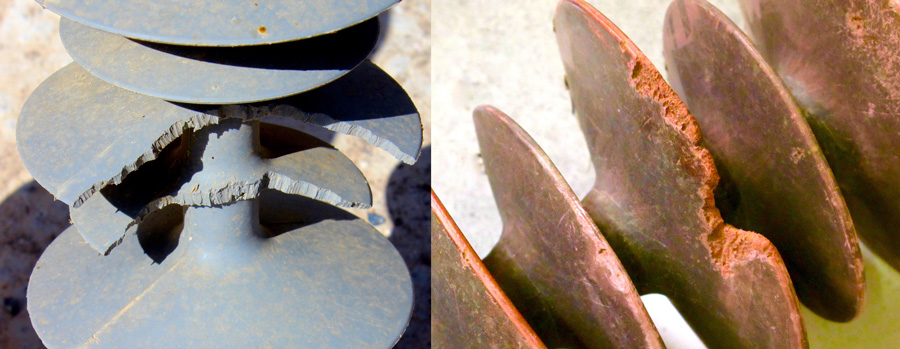
Cases of damage to composite insulators by rodent chewing or bird pecking have been reported and vary from small to large tears in sheds or even their complete removal and exposure of the core. Most such incidents have occurred on new insulators either during storage under less than ideal conditions or shortly after installation but before line energization. Evidence suggests that energizing the insulators does not necessarily eliminate all risk of such damage.
6. Shattering of Dielectric Shell
This type of failure applies only to glass disc insulators and is due to the instant release of the internal stresses induced in the shell during the toughening process. Vandalism by guns or rocks is a main cause and one of the reasons power supply companies in countries with such problems have been shifting from glass to composite insulators.
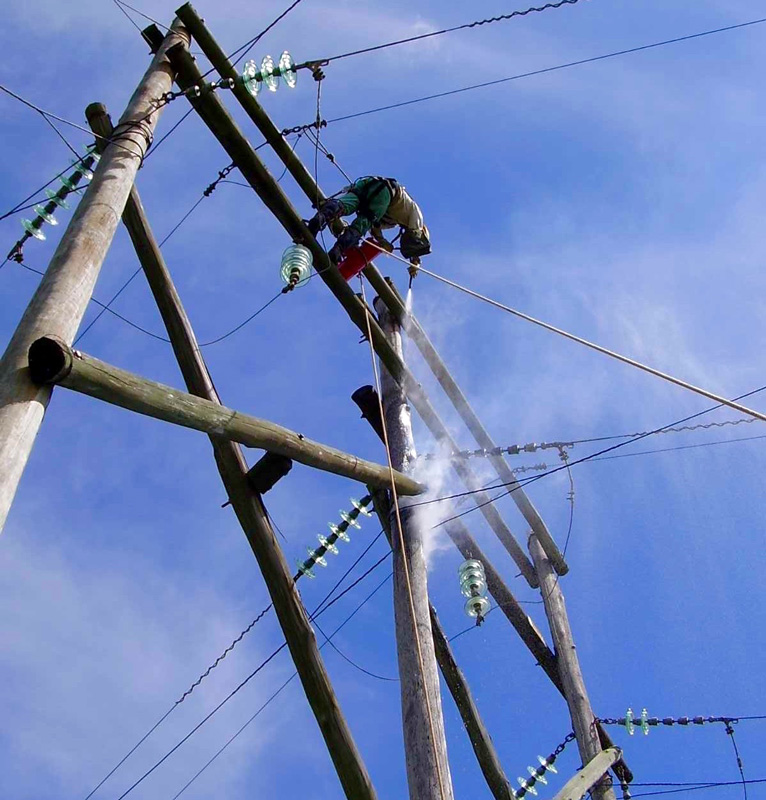
Sometimes this same failure occurs without any external agent and is referred to as ‘self or spontaneous shattering’. This phenomenon is specific to toughened glass and can be induced for example by poor quality control, evident in the presence of inclusions or impurities in the tensile zone of the glass body. Inclusions are hard particles that have not fully melted in the furnace and typically originate from the refractory bricks of the glass furnace or un-melted raw materials. Average size of such particles is less than 100 microns and they are not visible to the eye nor is there a direct and practical method to detect them. The most effective way to screen out toughened glass shells containing inclusions is application of specific thermal shocks to each glass shell prior to assembly of fittings. Such thermal shock tests are intended to weed out and destroy glass shells containing inclusions.
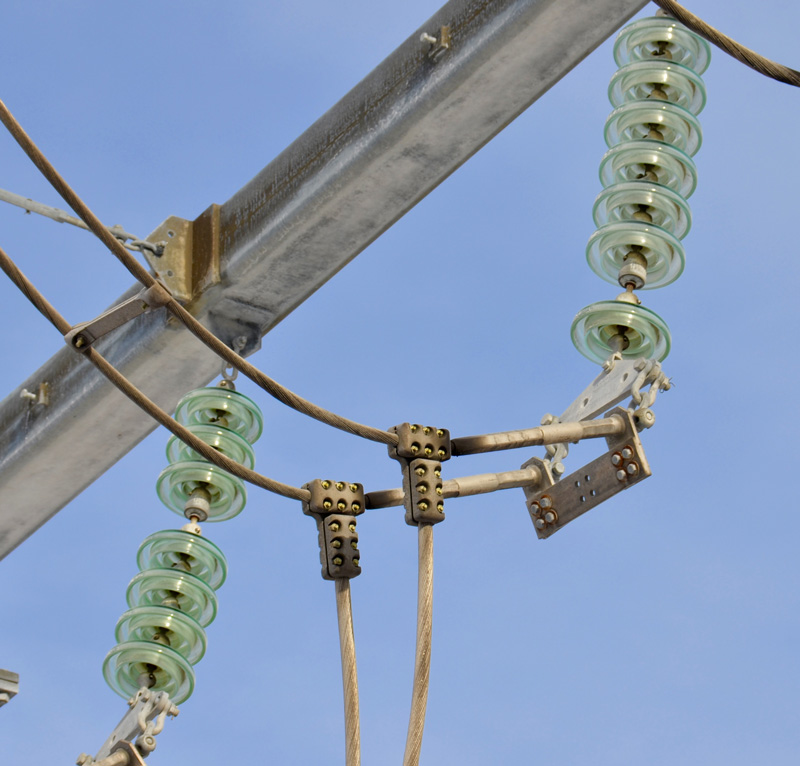
Even though thermal shock tests are carried out during production, some glass shells containing inclusions may survive this quality control measure and shatter during early years of service. Self-shattering can be triggered by a variety of external factors or might occur without any special circumstances. It has therefore been termed ‘spontaneous shattering’. Statistics reveal typical rates of spontaneous shattering on the order of 1 per 10,000 units per year or less, depending on manufacturer.
Cases have been reported where spontaneous shattering of glass discs have occurred on DC lines in a high pollution environment due to leakage current warming up the cement inside the fitting, causing it to expand slightly. The solution in such unusual cases was to apply RTV silicone coatings onto the discs to limit surface activity.
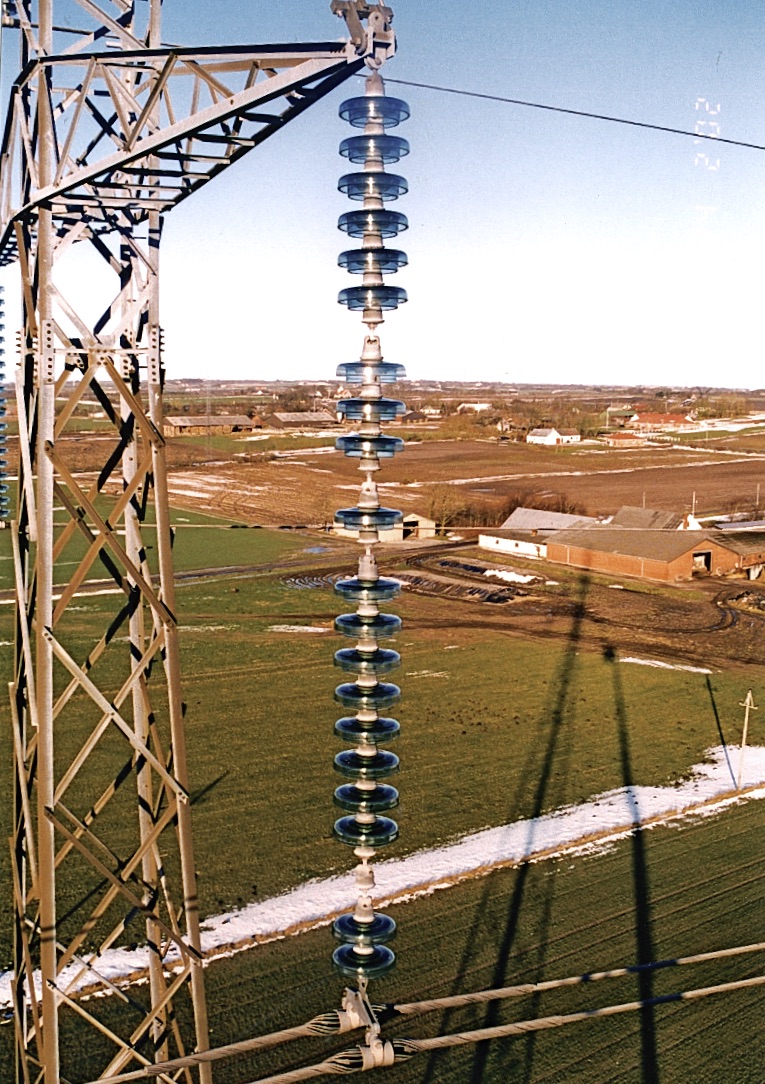
7. Radial Cracking
This type of failure has been attributed to cement growth phenomenon and applies only to porcelain insulators that were assembled using overly expansive Portland cement. Such radial cracking of porcelain disks can eventually result in internal puncture. There are no reliable statistics regarding the incidence of this type of failure but it is widely published that failure rates of porcelain insulators due to cement growth problems are generally associated with specific production batches.
8. De-Bonding at Critical Internal Interface
The quality of the critical interface between fiberglass rod and silicone rubber housing in a composite insulator has taken on renewed importance once investigations confirmed that the root cause of some recent failures was poor adhesion. While this was clearly revealed by non-standard adhesion tests, it was not when using existing IEC tests intended to verify integrity of such interfaces. Thus, quality is considered as not necessarily being fully covered by existing standards relating to such insulators.
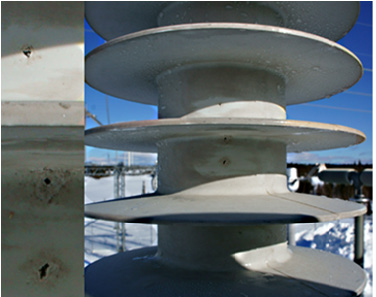
In 2008, key standards for composite line insulators (i.e. IEC 61109 and IEC 61952) were revised to align with the first edition of IEC 62217 – a standard which applies to all HV composite insulators and prescribes common tests including interface tests, housing tests, core tests, etc. IEC 62217 was last revised in 2012, with changes mainly in the UV ageing and the dye penetration tests. Moreover, both the wheel test and multiple stress tests were transferred to an IEC Technical Report. IEC 62217 has again been under revision and it can be considered that the IEC standards mentioned above utilized knowledge obtained a decade or so ago. For example, several tests in IEC 61109 have remained largely unchanged since their introduction in the first edition. These IEC standards are being reviewed, especially in light of recent field experience.
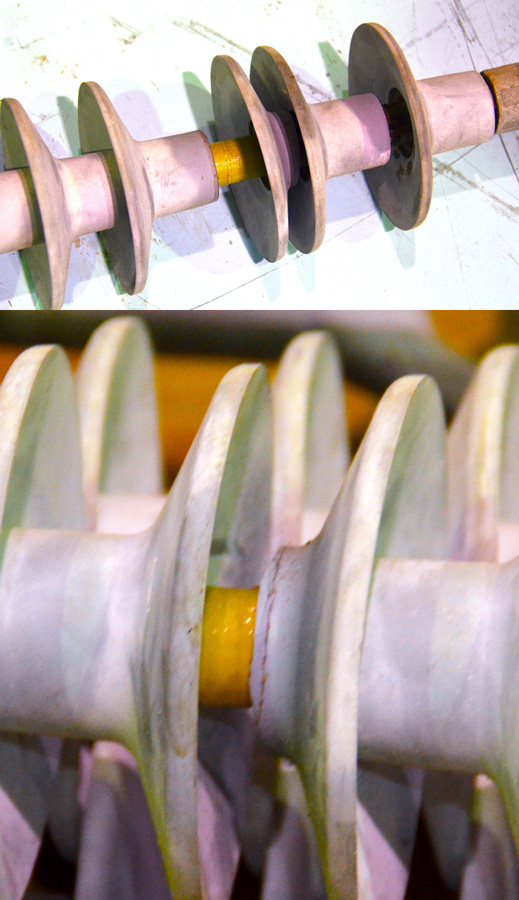
Discussion & Conclusions
It is more important to focus on mode of insulator failure and its impact on utility operations than on failure rates alone. This is because failure rate can depend on voltage class of the line. Users tend to specify only proven products on critical transmission lines and are more willing to experiment with potential new suppliers when it comes to lower voltage lines.
In the case where failure rate is defined as ‘mechanical separation of the string’, service experience clearly indicates that toughened glass insulator strings are significantly less prone to separation compared to strings of porcelain or composite insulators.
Failures that involve puncture of the dielectric body can be quite common for older porcelain insulators but are typically absent from toughened glass insulators and a rare occurrence in the case of composite insulators. Punctured discs cannot be identified from the ground and require additional testing equipment. Moreover, unless the puncture results in a short circuit it is not easily detectable.
Failures associated with cement growth problems (and consequent radial cracking) have been reported only on porcelain insulators and specifically because of the use of improper cement. These problems are hard to detect during the initial stages.
Risk of pin corrosion in areas of high pollution is a problem potentially affecting large populations of glass and porcelain disc insulators of older designs, before adoption of a sacrificial zinc sleeve countermeasure. When dealing with problems of vandalism and self-shattering rates of toughened glass insulators, it is important to consider that the glass stub typically remains electrically not punctured and the overall mechanical integrity of the insulator is retained as well. Strings with missing glass shell are also easily observed from the ground, although in countries with high vandalism, this can mean high maintenance costs. With regard to electrical performance, while a glass stub will have lost external insulating function, it will keep a possible arc in the air and outside the metal fittings with minimal risk of pin ejection and mechanical separation.
The organic nature of materials used for composite insulators gives rise to potential failure modes that are unique compared to ceramic insulators of porcelain or glass. For example, tracking and erosion of the housing material from surface discharges, pecking by birds, tracking of the fiber glass core and brittle fracture are all potential modes of failure. Despite the increased number of possible failure modes, composite insulators have improved significantly. Today, well designed and properly manufactured such units offer excellent proven service performance, even under demanding service conditions, combined with very low rates of failure.